Introduction to Metabolic Processes
Hello dear readers,
Before we dive into today’s content, I want to share something important with you. Although I’m passionate about the topic we’re going to discuss and have spent a lot of time researching and learning about it, it’s essential to clarify that I am not a professional in the field.
What you will read next are insights and information that I have gathered based on my personal experience and self-taught studies. My intention is to start a conversation, share what I’ve discovered, and maybe help someone who is on the same journey of discovery. If you are an expert on the subject, your comments and guidance are very welcome, as we are all here to learn and grow together.
Now, let’s get to the good stuff!
Metabolic processes are fundamental biological mechanisms through which living organisms convert nutrients into usable energy. These processes are crucial for maintaining life, as they provide the energy required for various cellular activities. At the core of metabolism is cellular respiration, a multi-step pathway that cells use to extract energy from organic molecules. During this process, the energy stored in chemical bonds of nutrients, like glucose, is released and harvested for cellular functions.
There are two primary types of metabolic processes: aerobic and anaerobic. Both play essential roles in energy production, but they operate under different conditions and yield varying amounts of energy. Aerobic processes require oxygen and are typically more efficient, extracting a greater amount of energy from nutrients. Anaerobic processes, on the other hand, do not require oxygen and are generally less efficient, producing less energy per molecule of nutrient consumed.
Understanding the distinction between these metabolic pathways is not just academic; it has practical implications in fields ranging from medicine to athletic training. For instance, aerobic metabolism is predominantly used during prolonged, moderate-intensity activities, such as jogging or swimming. Conversely, anaerobic metabolism becomes the primary source of energy during short bursts of high-intensity activities, like sprinting or weight lifting.
Given their significance, it’s essential to delve deeper into the mechanics of both metabolic processes to comprehend how they contribute to overall energy production. This knowledge can inform everything from nutritional planning to the development of medical treatments. As we explore further, the details of these processes will illuminate how they are tailored to meet the energy demands of various organisms and activities.
What is Aerobic Respiration?
Aerobic respiration is a fundamental biological process that facilitates energy production in cells through the use of oxygen. This intricate mechanism involves several stages, each contributing to the efficient conversion of glucose and oxygen into adenosine triphosphate (ATP), the energy currency of the cell. The process of aerobic respiration can be broken down into three key stages: glycolysis, the Krebs cycle (also known as the citric acid cycle), and the electron transport chain.
Glycolysis is the initial stage of aerobic respiration and occurs in the cytoplasm of the cell. During glycolysis, one molecule of glucose is enzymatically broken down into two molecules of pyruvate. This process yields a net gain of two ATP molecules and also results in the production of reduced nicotinamide adenine dinucleotide (NADH), which carries electrons to later stages of respiration.
Following glycolysis, the pyruvate molecules are transported into the mitochondria, where the Krebs cycle takes place. This cycle involves a series of enzyme-catalyzed reactions that further oxidize the pyruvate to carbon dioxide. In addition to generating two more ATP molecules, the Krebs cycle produces high-energy electron carriers, including NADH and flavin adenine dinucleotide (FADH2), which are crucial for the final phase of aerobic respiration.
The electron transport chain, located in the inner mitochondrial membrane, is the final and most ATP-productive stage of aerobic respiration. Here, the electrons carried by NADH and FADH2 are transferred through a series of protein complexes. This transfer drives the pumping of protons across the membrane, creating a proton gradient. As protons flow back through ATP synthase, a significant amount of ATP is synthesized, with aerobic respiration typically generating a total of about 30-32 ATP molecules per glucose molecule metabolized.
Aerobic respiration occurs in conditions where oxygen is abundant. Its high efficiency in ATP production makes it an indispensable process for energy-demanding activities within an organism. The presence of oxygen as a final electron acceptor enables the complete oxidation of glucose and the maximal extraction of energy, distinguishing aerobic respiration from its anaerobic counterpart.
Anaerobic respiration is a metabolic process that occurs in the absence of oxygen. Unlike aerobic respiration, which relies on oxygen to produce energy, anaerobic respiration uses other electron acceptors to sustain cellular functions. This process is primarily employed by organisms or cells when oxygen is scarce or unavailable.
There are several types of anaerobic respiration, with two of the most well-known being lactic acid fermentation and alcoholic fermentation. Lactic acid fermentation occurs in muscle cells during intense exercise. When the demand for ATP (adenosine triphosphate) exceeds the oxygen supply, muscles switch to anaerobic respiration, converting glucose into lactic acid and yielding a small amount of ATP. This lactic acid buildup is often responsible for the sensation of muscle fatigue and soreness post-exercise.
Alcoholic fermentation, on the other hand, is prevalent in yeast and some bacteria. This type of anaerobic respiration converts glucose into ethanol and carbon dioxide, producing a limited amount of ATP. This process is utilized in brewing, baking, and ethanol production, showcasing its industrial significance.
While both types of anaerobic respiration allow organisms to produce ATP in the absence of oxygen, they are significantly less efficient compared to aerobic respiration. Anaerobic processes typically generate only 2 ATP molecules per glucose molecule, whereas aerobic respiration can yield up to 36-38 ATP molecules per glucose molecule. This stark contrast in efficiency explains why cells only resort to anaerobic respiration under specific conditions, such as oxygen depletion during strenuous activity or in anaerobic environments where oxygen is entirely absent.
In conclusion, anaerobic respiration is a critical survival mechanism that enables organisms to continue producing energy when oxygen is not available. However, its inefficiency compared to aerobic processes underscores the importance of oxygen in energy production. Understanding the nuances of anaerobic respiration not only sheds light on cellular physiology but also has far-reaching implications in various scientific and industrial applications.
Key Differences Between Aerobic and Anaerobic Processes
Understanding the distinctions between aerobic and anaerobic processes is crucial when examining cellular respiration and energy production in organisms. These processes fundamentally differ in several aspects, including oxygen dependency, energy output, byproducts, and operating conditions.
1. Oxygen Usage:
– Aerobic Processes: Require oxygen. Oxygen acts as the final electron acceptor in the electron transport chain, facilitating the complete oxidation of glucose molecules.
– Anaerobic Processes: Do not require oxygen. These processes are utilized when oxygen is scarce, relying instead on either internal or external agents for electron acceptance.
2. Energy (ATP) Yield:
– Aerobic Processes: Generate a significantly higher yield of adenosine triphosphate (ATP), typically producing about 36 to 38 ATP molecules per glucose molecule metabolized. This efficiency stems from fully oxidizing glucose to carbon dioxide and water.
– Anaerobic Processes: Yield considerably less ATP, generally producing only 2 ATP molecules per glucose molecule. The limited energy output is due to incomplete glucose breakdown.
3. Byproducts:
– Aerobic Processes: Produce carbon dioxide and water as primary byproducts. These substances are non-toxic and easily expelled from the body.
– Anaerobic Processes: Create different byproducts, depending on the organism and specific pathway. For example, lactic acid is produced in human muscles under anaerobic conditions, while ethanol and carbon dioxide are produced by yeast during fermentation.
4. Conditions:
– Aerobic Processes: Occur in environments where oxygen is plentiful, such as the mitochondrial matrix in eukaryotic cells.
– Anaerobic Processes: Operate in oxygen-deprived conditions, such as waterlogged soils, deep-sea sediments, or during intense exercise in muscles.
This comparative analysis highlights the inherent differences between aerobic and anaerobic processes. By understanding these distinctions, one can better appreciate how cells efficiently produce energy under varying environmental conditions.
Physiological Impact on the Human Body
Aerobic and anaerobic processes have distinct physiological impacts on the human body, influencing everything from cardiovascular health to muscle strength and endurance. Aerobic exercise, such as running, cycling, or swimming, relies heavily on oxygen intake to sustain prolonged physical activity. This type of exercise significantly improves cardiovascular health by enhancing the efficiency of the heart, lungs, and circulatory system. Regular engagement in aerobic activities strengthens the heart muscles, lowers blood pressure, and increases the body’s ability to use oxygen. These benefits culminate in improved endurance, allowing individuals to perform activities for longer periods without fatigue.
On the other hand, anaerobic exercise includes activities like weight lifting, sprinting, and high-intensity interval training. Unlike aerobic processes, anaerobic exercises are intense and performed in short bursts, not relying on oxygen as the primary energy source. Instead, these exercises utilize glycogen stored in muscles. Anaerobic activities are incredibly effective for increasing muscle strength, power, and muscle mass. They also aid in developing speed and agility, which are crucial for activities requiring quick, explosive movements.
Each type of exercise has its benefits and limitations. Aerobic exercises are beneficial for overall cardiovascular health, weight management, and improving endurance. However, they might not be as effective for building muscle strength and mass. Conversely, while anaerobic exercises are excellent for muscle building and improving strength and power, they may not enhance cardiovascular health to the same extent as aerobic exercises. Additionally, excessive engagement in anaerobic exercises without proper rest can lead to muscle fatigue and overuse injuries.
Therefore, a balanced workout regimen that incorporates both aerobic and anaerobic exercises is essential for optimal health and fitness. This combination can ensure comprehensive benefits including enhanced cardiovascular health, improved muscle strength, and overall physical performance.
Environmental and Industrial Applications
Understanding aerobic and anaerobic processes is integral to numerous environmental and industrial applications. These processes are leveraged for their distinct biological mechanisms and outcomes, offering tailored solutions for specific needs.
In wastewater treatment, aerobic and anaerobic bacteria play indispensable roles. Aerobic treatment systems introduce oxygen to support the metabolism of aerobic bacteria, which consume organic matter, resulting in carbon dioxide, water, and biomass as by-products. These systems are highly efficient for reducing biological oxygen demand (BOD) and are widely used in municipal and industrial wastewater treatment facilities. Conversely, anaerobic treatment systems create an oxygen-free environment, facilitating the activity of anaerobic bacteria. These bacteria convert organic material into biogas, primarily methane and carbon dioxide, while producing considerably less biomass. The biogas generated can be harnessed as a renewable energy source, making anaerobic treatments highly sustainable and cost-effective for waste management.
In composting and decomposition, both aerobic and anaerobic processes are pivotal. Aerobic composting relies on oxygen-dependent microbes to decompose organic waste, producing humus—a nutrient-rich soil amendment. This process is rapid and results in minimal odor, making it suitable for community and commercial composting operations. Anaerobic decomposition, in contrast, is slower and occurs in oxygen-deprived conditions. While it is less common for traditional composting due to the potential for odor, it offers advantages in closed-system applications where biogas can be captured and utilized.
Industrially, these biological processes are harnessed in various ways. The brewing industry employs controlled anaerobic fermentation by yeast to convert sugars into alcohol and carbon dioxide, essential for producing beverages like beer and wine. Similarly, biofuel production capitalizes on anaerobic digestion to transform organic material into biogas, which can be purified and used as an energy source. Aerobic processes are also used in bioremediation to detoxify contaminated environments by encouraging aerobic organisms to break down hazardous substances into harmless by-products.
Thus, the strategic application of aerobic and anaerobic processes has far-reaching implications, enhancing our ability to manage waste sustainably, create valuable by-products, and address environmental challenges decisively.
Real-World Examples and Case Studies
Aerobic and anaerobic processes are foundational to many real-world scenarios, showcasing their practical applications across diverse fields such as ecosystems, industrial operations, and medical scenarios.
In natural ecosystems, the decomposition of organic matter provides a revealing example of both processes at work. Forest ecosystems, for example, rely heavily on aerobic respiration facilitated by bacteria and fungi. These microorganisms feed on fallen leaves and dead plants, breaking them down in the presence of oxygen and converting them into simpler substances, which, in turn, enrich the soil. This process is critical for nutrient cycling and maintaining soil health.
Conversely, wetland environments often demonstrate anaerobic processes, where oxygen levels are low. Here, the breakdown of organic matter occurs under anaerobic conditions, facilitated by different bacterial communities. These microorganisms engage in processes like methanogenesis, where methane is produced as a byproduct of organic degradation. Wetlands, therefore, act as significant natural sources of methane, influencing global greenhouse gas levels.
In the realm of industrial operations, wastewater treatment plants provide a practical illustration of aerobic and anaerobic processes. Aerobic treatments, involving activated sludge systems, are designed to accelerate the digestion of organic pollutants using aerobic bacteria. These systems require the continuous supply of oxygen to sustain the microbial activity, thereby efficiently breaking down contaminants. Anaerobic digesters, on the other hand, are employed to treat sludge or highly concentrated industrial effluents. Such digesters utilize anaerobic bacteria to decompose organic matter in the absence of oxygen, producing biogas—a mixture of methane and carbon dioxide—that can be harnessed for energy production.
From a medical perspective, the human body leverages both processes for optimal functioning. Aerobic respiration is a critical component of cellular metabolism, ensuring that our cells receive enough energy to perform vital functions. Conversely, under strenuous conditions where oxygen supply may be limited, muscles can switch to anaerobic respiration, resulting in the production of lactic acid and quick bursts of energy. This adaptation is vital for high-intensity activities, although prolonged anaerobic activity can lead to muscle fatigue.
These examples elucidate the significance of understanding aerobic and anaerobic processes. Whether in natural ecosystems, industrial applications, or human physiology, these processes underpin essential functions and offer numerous practical benefits and challenges.
Conclusion and Future Perspectives
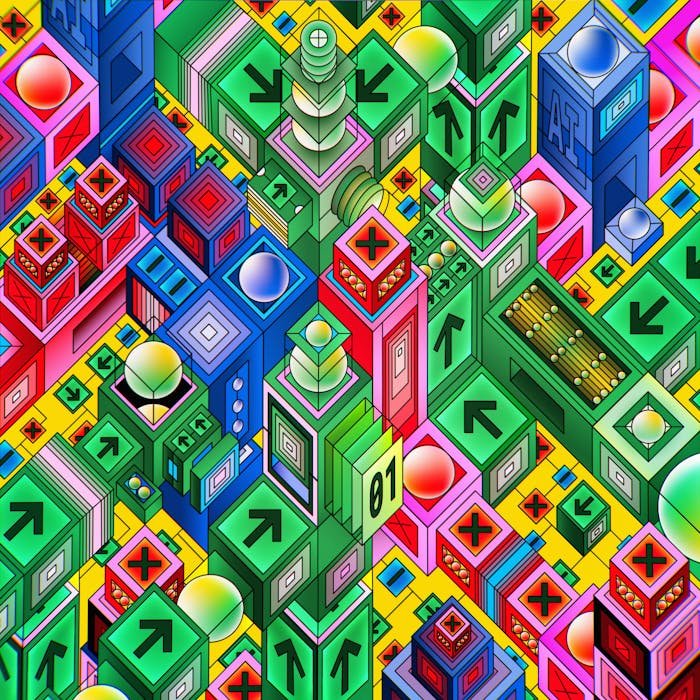
Understanding the fundamental differences between aerobic and anaerobic processes is pivotal for various scientific disciplines and practical applications. Aerobic processes, which require oxygen to produce energy, are notably efficient and are fundamental to many organisms, including humans. These processes are crucial for cellular respiration and are essential in higher organisms for survival and function. On the other hand, anaerobic processes, which do not require oxygen, are employed by certain microbes and serve as alternative pathways for energy production, especially under low-oxygen conditions.
The distinction between these processes lies in their biochemical pathways, energy yields, and environmental conditions. While aerobic respiration results in higher energy output and the complete oxidation of glucose, anaerobic respiration or fermentation processes yield less energy and produce byproducts such as lactic acid or ethanol. Recognizing these mechanisms’ distinct characteristics is essential for optimizing various industrial and environmental applications, such as waste treatment, biofuel production, and medical therapies.
Future research will likely delve deeper into leveraging both aerobic and anaerobic processes for innovative solutions. For instance, combining these processes could enhance bioenergy production efficiency, given the complementary nature of their mechanisms. Advances in genetic engineering could also lead to the development of microorganisms with optimized pathways for specific industrial applications, potentially revolutionizing waste management and renewable energy sectors.
Additionally, ongoing research into the interplay between these processes in natural ecosystems could provide insights into maintaining environmental homeostasis and biodiversity. Understanding how organisms switch between aerobic and anaerobic metabolism in response to environmental changes can inform conservation strategies and ecosystem management.
In sum, the dual study of aerobic and anaerobic processes offers a promising horizon for scientific innovation and practical solutions to pressing issues. Continued exploration and technological advances in this field are likely to yield more sustainable and efficient methods of energy production and environmental management, impacting numerous aspects of modern life and ecological balance.